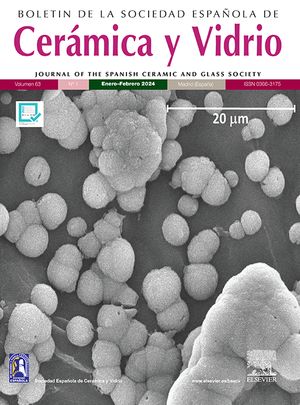
Manuscripts on Advanced Ceramics dedicated to the memory of Professor Víctor M. Orera
More infoThe aim of this study is to describe the steps and complications encountered in the design, fabrication and calibration of ceramic passive samplers for the analysis of water contaminants. A ceramic passive samplers consists in a porous ceramic tube where a polymeric sorbent is placed in the inner side to preconcentrate the analytes from water with high enrichment factors. The diffusivity and uptake of contaminants depend on the ceramic porous structure that influences the sampling rate. Although the concept stands up for its simplicity, in real life passive samplers face several problems: fouling, clogging and saturation and then the uptake of contaminants may not be linear during the deployment period. With ceramic passive samplers these problems can be solved by optimizing the ceramic structure and porosity. Over eight year period, we have had a fruitful collaboration with Prof. Victor Orera, aimed to develop customized ceramic membranes to unselectively accumulate the contaminants present in different types of water with the overarching objective to monitor chemical pollution present in the environment. The use of customized ceramic passive samplers (CPS) will permit to identify main compounds in water, their evolution in time, identify the sources of pollution and finally gather enough knowledge and data to effectively propose control and remediation actions. In this study, we describe the steps undertaken in the development of ceramic samplers, their characteristics, the calibration procedure used, their performance and applicability. The work herein presented has been possible due to the brilliant ideas, enthusiasm, perseverance and motivation of Prof. Orera, but also because his joyful and cheerful character made this collaboration really pleasant and valuable.
El objetivo de este estudio es describir las etapas y complejidades halladas en el diseño, fabricación y calibración de muestreadores pasivos cerámicos para el análisis de contaminantes en agua. Los muestreadores pasivos cerámicos consisten en un cilindro cerámico poroso donde se coloca un adsorbente polimérico en el interior que permite preconcentrar los analitos del agua con elevados factores de enriquecimiento. La difusividad y la adsorción de contaminantes dependen de la estructura porosa de la cerámica que influye en la velocidad de muestreo. Aunque el concepto destaca por su simplicidad, en la vida real el uso de los muestreadores pasivos conlleva varios problemas: colmatación por partículas y materia orgánica, obstrucción y saturación, de manera que la adsorción de contaminantes puede no ser lineal. Con los muestreadores pasivos cerámicos, estos problemas se pueden resolver optimizando la estructura y la porosidad de la estructura cerámica. Durante un período de ocho años hemos tenido una fructífera colaboración con el Prof. Víctor Orera con el objetivo de desarrollar membranas cerámicas «a medida» para acumular de manera no selectiva los contaminantes presentes en distintos tipos de agua. El uso de muestreadores pasivos cerámicos permite identificar las fuentes de contaminación, los contaminantes más ubicuos y su evolución en el tiempo. La monitorización de la calidad del agua de forma sistemática mediante muestreadores pasivos proporciona nuevos datos que permiten proponer acciones de remediación de manera efectiva. En este artículo se describen los pasos realizados en el desarrollo de los muestreadores cerámicos, pretende destacar sus propiedades y el procedimiento de calibración, así como su rendimiento y aplicabilidad. Este estudio ha sido posible gracias al conocimiento, el entusiasmo, la perseverancia y la motivación del Prof. Orera, pero también por su carácter alegre y optimista que hizo esta colaboración realmente grata y valiosa.
Environmental monitoring programs require the use of efficient and reliable sampling and analytical techniques. Sampling methods need to be representative of a water body and should be simple to use and to implement. Currently, grab sampling is the most common way to collect samples from surface waters although it might not be completely representative due to time dependent results. Passive samplers (PS) for the analysis of trace substances in waters have been extensively developed in recent years [1]. They can be used to better evaluate the presence of contaminants in the aquatic environment and have emerged as an alternative to grab sampling as provide representative information on the contaminants present in water [2]. Main advantages are: (i) in situ uptake of the bioavailable fraction of contaminant; (ii) time integrated concentrations; (iii) low cost; (iv) ease of deployment, making multi-site screening possible; and (iv) high enrichment factors and reduced matrix interferences when deployed in the field. Passive samplers are in particular useful in areas where concentration of the contaminants is not constant or in situations where an integrated measurement is needed. Passive samplers, in their various formats [3], are nowadays a key asset for environmental monitoring and regulatory compliance [4].
Ceramic passive samplers (CPS) were first developed by Martin et al. for the time-integrated monitoring of water pollutants [5]. They consist in a ceramic cylindrical membrane that acts as a diffusion barrier and allows the free flow of contaminants from the media to the receiving phase due to the difference in chemical potentials between the external and internal area of the sampler [6]. Then, contaminants are retained in a sorbent placed inside the sampler which is thereafter analyzed usually using mass-spectrometric based techniques to allow the identification of a chemical family [7] or multiple contaminants [8]. The efficiency and performance of the CPS is calculated by measuring the diffusivity (De) of contaminants in controlled laboratory conditions [9–10]. The higher the diffusivity, the higher the sampling rate (Rs) and the higher the mass of a compound accumulated in the sorbent, thus, the capacity to detect contaminants from water. The diffusivity of contaminants depends on the characteristics of the ceramic membrane, where porosity, inertness, dimensions, pore size and wall thickness are the key parameters to ensure high enrichment factors as a function of time [11]. Porosity has to be large enough in order to maximize the contained water volume in the membrane, and high connectivity between pores enhances high flow rates. In addition, pore size has to be small enough to allow capillarity forces to keep the water layer inside the ceramic walls avoiding swelling effects [12].
CPS first developed by Martin et al., the so called ceramic dosimeter, have a configuration of 50mm of length, 1.5mm of wall thickness and 10mm outer diameter and a pore diameter of 5nm [6,13]. They were proven useful for monitoring water contaminants in groundwater for long periods of time [14]. In these samplers, the flow of contaminants through the ceramic wall and the accumulation in the receiving phase depend on the type and nature of water being monitored, for instance, clogging may occur because of the small pore size when sampling waters with high organic matter or particle content (e.g. wastewaters). Therefore, the porosity and dimensions can be adapted or customized to have a higher porosity and concomitantly, high Rs.
In 2012, we started an altruistic but fruitful collaboration with Prof. Orera aiming at optimizing the structure of ceramic passive samplers for the integrated monitoring of contaminants in a customized way for being applied in different types of waters. The main difference compared to previous ceramic dosimeters is a high connected porosity and pore morphology consisting of large pore cavities connected by smaller pores. This porous ceramic material has a density and a specific pore diameter that causes chemicals to flow freely through the ceramic membrane which has high resistance. The idea is to allow for a high water volume inside the ceramic support. We developed a range of ceramic membranes with different pore structure that enabled the modulation of contaminant uptake. The ceramic wall was designed to support a high total porosity without deteriorating the mechanical integrity of the membrane, so that organic contaminants (with molecular size of generally angstroms) could diffuse across the wall at different rates. The structure was also designed so that the inner sorbent (diameter>30μm) did not escape from the cylinder and that the amount inserted could also be adapted to have high capacity for flexible deployment periods. However, the development of such structures was not a straightforward procedure as we confronted several challenges.
Based on the work we have carried out during the past 8 years, the aim of the present study is to describe the procedures developed but also to highlight the complications and drawbacks faced in the design and fabrication of ceramic passive samplers. We highlight the performance of the actual passive samplers developed. Our work stands-up basically because of the energy, interest and ambition of Prof. Orera who fought and perseverated to promote easy-to-implement monitoring procedures using passive samplers in an efficient, cost-effective and with a simple deployment procedure. At present, the designed ceramic passive samplers are at TRL 6–7, they have been validated for field deployment throughout the project IMAQUA (https://eurecat.org/en/portfolio-items/imaqua/) and have been proposed as monitoring tools in 2 European Union projects of the Greendeal call.
Design of the structureThe performance of the passive samplers depends essentially on their dimension, and it is needed to optimize the structure in order to have a high diffusivity and uptake of contaminants. Based on the equations that rule the diffusivity across a ceramic layer, the dimensions and porosity were optimized in order to have a sensitive system capable of trace level determination of contaminants in water. The equations herein indicated are developed and demonstrated in previous studies [1,5].
Diffusion parameter De (Eq. (1)) is related to the actual diffusion coefficient in water Dw according to the membrane pore geometry that depends on the effective or connected porosity ɛ, the constriction factor δ, and the tortuosity factor τ[12].
δ is assumed to be 1 as molecules will diffuse through large channels with more probability than in between the pore walls. In practice, to have a high diffusivity to allow a high sampling rate, it is important to have a high ɛ and low τ, which is obtained by optimizing the amount of porogen used in the fabrication of the ceramic membrane.
Once a sorbent is placed inside the ceramic membrane, the mass of contaminants (M) accumulated in the sorbent depends on De but also on the concentration in water (Cw), the time deployed (t) and the dimensions of the sampler. The higher the exposure area (A) and the smaller the diffusion layer thickness (L) of the sampler, the larger the amount accumulated (Eq. (2)).
Thus, different ceramic membranes were optimized in a size large enough to have a high diffusivity but without affecting its integrity (breakability). In our case, different dimensions were tested (Fig. 1), where mainly the following considerations were taken into account:
- -
Size: we started with the dimensions of the ceramic passive sampler previously proposed [6,13] and were adapted to allow different configurations for diverse purposes.
- -
Breakability: a thin wall makes the sampler very brittle and this affects set-up and deployment.
- -
Fragility: a very porous membrane with high connected porosity, large porous or high porous density makes the structure fragile. In addition, porous larger than 30μm would allow that the sorbent placed inside the ceramic membrane would escape.
- -
Inner volume: if the outer diameter increases to increase surface area, and also does the inner diameter to be consistent with an L as small as possible (without being breakable), the inside cavity is too large and thus more sorbent needs to be deployed. Considering the price of most bulk sorbents (600–1000€/100g), it is important to have a dimension so that the inner volume can accommodate the smallest amount of sorbent (e.g. 200–500mg which is used in solid-phase extraction cartridges) but with enough capacity to retain water contaminants.
- -
Material: ceramic membranes were made of alumina as this material allows the formation of porous structures at high temperature and is highly resistant. However, upon deployment, it was observed that alumina is hydroscopic and a layer of bubbles is formed upon the wall, and could affect contaminants to diffuse in. As trial, samplers were also prepared of chalk to test diffusivity, however, chalk dissolved in water when deployed for >1 week.
The dimensions offering higher diffusivity and physical strength [12] were estimated and fabricated as indicated below.
Fabrication methodWith the dimensions and morphology optimized, the passive samplers were fabricated by slip casting method using alumina powder (grain size of 0.24–0.49μm) and water (60–80% in volume), corn flour as porogen agent (12–25% volume) and Duramax D-3005 as dispersant (1% in solid weight) to stabilize the suspension. The prepared mixture is poured in a plaster mold with the desired geometry, and it is kept between 10 and 20min. If a thinner structure is required, permanence on mold times can be reduced to 6min. The time that the suspension remains in the mold determines the thickness of the raw piece, and the composition of the suspension determines its porosity and microstructure. After casting the green membranes, they were pre-sintered in air at 950°C for 4h. The membranes were then sintered at 1300°C for 3h dwelling time and heating and cooling rates of 4°Cmin−1 to obtain high stiffness and mechanical resistance, also providing high porosity with porous size homogeneity (Fig. 2A). The microstructure of the porous ceramic was first studied with an optic microscope and then in polished transverse membrane cross-sections using a JEOL 6301F scanning electron microscope (SEM) and the homogeneity in the structure was evaluated according to a procedure previously published [6]. Open and connected porosity was measured by means of an Hg porosimeter (Poremaster, Quantachrome; maximum pressure 30,000psia). In these measurements, the intruded mercury volume is obtained as a function of the liquid (mercury) pressure. Total porosity was determined by gravimetric density measurement and depending on the structure ranged from 35 to 50% in fair agreement with the volume of sacrificial phase indicating that most of pore volume is produced by pore former phase. Fig. 2B shows the pore size homogeneity of one the developed structures with pore sizes between 300 and 1000nm.
Fig. 3A and C shows a typical SEM image of a ceramic membrane transverse cross-section where both corn flour and graphite pore formers were used for a low porous structure (CPS 1, 40% porosity) and a highly porous one (CPS 5, 50% porosity). A homogeneous and continuous distribution of spheroid cavities around 10μm diameter through all the membrane depth is observed (Fig. 3B). Fig. 3D shows the detailed image of the microstructure. Effect of partial sintering on alumina microstructure can be seen. Polygonal alumina grains indicate that strong struts have been produced but leaving intergranular porosity. This porosity is a consequence of partial sintering and it is related with the alumina grain size being 2–5 times smaller than alumina grains in agreement with conventional sintering theories [6].
Calculation of the diffusivity using methylene blueCalibration of passive samplers is commonly performed with target compounds to be analyzed and using a high concentration gradient to enhance the amount accumulated [1]. However, calibration using target compounds is time consuming and expensive, especially if mass spectrometric techniques are used for analysis. Therefore, we came up with the idea to use methylene blue (C16H18ClN3S) to determine the flow and diffusion rates of the different samplers and to test permeation, equilibrium and saturation. Important was that the diffusivity of the different samplers can be compared using this simple test procedure. Methylene blue is cheap, non-toxic and easy to determine spectrophotometrically. The procedure used was as follows: a beaker with 4L of mineral water is dosed with methylene blue at 10mg/L (Fig. 4A). Ceramic membranes are filled with clean mineral water, closed with the caps, placed in a support made of net and then placed inside the methylene blue solution. In this step, no adsorbent is used inside the CPS as the membrane diffusivity will be first evaluated. During the exposure period, the beaker is placed in an orbital shaker (we used a KS500, Janke&Kunkel, IKA WERK, Staufen, Germany) with a constant temperature (22–23°C) and covered with aluminum foil to avoid degradation. The orbital shaker has a circular shaking motion at low speed (100rpm), which does not create vibrations nor produce heat, and it was used to induce a liquid movement simulating the circulating water in a river or wastewater. Diffusivity was also tested without shaking. Methylene blue is measured with a spectrophotometer HP-Agilent 8453 (Agilent Technologies, CA, USA) with a diode array detector (DAD) at a wavelength of 664nm which is the maximum absorbance (Fig. 4B). To quantify the concentration of methylene blue in both the inner passive sampler and the external solution, a calibration curve over the concentration range of 1–10mg/L was also built (Fig. 4C). In fact, the concentration of the external solution was monitored to determine the stability of methylene blue throughout the deployment time (Fig. 4D).
Calibration of ceramic passive samplers with methylene blue indicating: (A) the vessel spiked with methylene blue at 10mg/L with the samplers inside; (B) UV spectra of methylene blue where different colors indicate different concentrations in the calibration curve and in the tests performed; (C) calibration curve over a concentration range of 1–12mg/L that is used to calculate the concentration and diffusivity; (D) stability of methylene blue in water over the period of 15 days during which calibration is performed and (E) calibration curves obtained for 5 passive samplers ranging from low (CPS 1) to high porosity (CPS 5).
The methylene blue concentration inside the membrane was measured as a function of the deployment time (see Fig. 4E). The deployment time depends on the type of ceramic membrane, as very porous ones will reach saturation in little time whereas the less porous will take more time for methylene blue to diffuse in to reach equilibrium with the surrounding media. Thus, the calibration has to include a period of time long enough to observe the diffusion process up to saturation. This calibration is done in duplicate measurements and preliminary tests for a period of half month are recommended to determine the uptake dynamics of the ceramic structure. In practice, two cylinders are removed after 1, 2, 5, 6, 9, 12 and 15 days. The optical absorption of the inner solution is measured spectrophotometrically and the concentration of methylene blue calculated based on the calibration curve. Based on this time-dependent calibration, the diffusivity of methylene blue was calculated as follows (Eq. (3)):
where k is the rate constant (concentration–time slope) of the calibration (Fig. 4E) and depends on the dimensions of the passive sampler. According to Eq. (3), a bigger pore size and pore density results in higher diffusivity toward the inner cavity by chemical gradient. In addition, a high porosity and concomitant higher De will avoid fouling effects when deployed in surface or wastewaters waters. This means that the system will offer high enrichment factors and good sensitivity for monitoring organic pollutants in waters for relatively long periods. As other ceramic dosimeters, the device is flow-independent and permits the sampling of environmental waters as diffusion constant through the wall will not be affected by the water flow.Therefore, methylene blue calibration permits to compare the uptake dynamics of different passive samplers and determine the time until saturation is reached and this information is useful to define deployment conditions as a preliminary step before calibration with individual contaminants.
Calibration with contaminants to be analyzedBecause the diffusivity of the contaminants across the ceramic membrane depends on their polarity and solubility, the calibration has to be done for each single compound to be analyzed. Passive samplers are calibrated to calculate k, Rs and the effective De. Rs (Lday−1) is a term commonly used in the field of passive samplers and corresponds to the amount of analyte retained by the sampler per unit of time at constant concentration in the surrounding media (the difference in chemical potentials are constant) (Eq. (4)); the Rs per receiving phase mass unit (g) is the uptake rate (Lday−1g−1) [15] and considers the dimensions of the sampler (Eq. (5)).
Recently, it has been indicated that predicting the sampling rate with compound properties is not reliable and should be determined experimentally to estimate the concentration of target compounds in water [16]. Several calibration methods that exist for passive sampling include equilibrium extraction, linear uptake, and kinetic calibration [7,17]. The passive samplers herein developed follow a linear uptake over periods of >15 days, although some of them show an increase and thereafter the uptake decreases as in a convex function. This is related to the stability of contaminants in water, and therefore the deployment procedure that we suggest is to determine the quantity adsorbed into the sorbent and in parallel to quantify the concentration of compounds present is solution each time a sampler is removed as compound stability affects the calibration procedure.
The laboratory calibration of passive samplers for a set of organic contaminants is a step-by-step procedure where the following is to be done:
- -
Samplers should be filled with the desired sorbent according to the compounds to be analyzed [18].
- -
Fill a glass beaker with a volume of water (e.g. 4L minimum) so that it has enough capacity to submerge the samplers. Bottled water is recommended at first instance as its pH and mineral content better simulates natural waters, compared to Milli-Q water.
- -
Spike the water in the beaker with the mixture of test standards at concentrations of 20μgL−1. This concentration is chosen as it can be easily in water.
- -
Enmeshed the samplers in a support (either tulle mesh or aluminum) that does not pollute the water nor release any compound (e.g. plasticizers if plastic is used or iron if tin structures are used). The samplers should not be in contact with one-another nor with the wall of the beaker as this would affect diffusivity.
- -
Insert the enmeshed passive samplers in the beaker and place the beaker in an orbital shaker for the period of time that calibration is performed. During exposure period, the temperature needs to be kept constant (e.g. room temperature at 22–23°C) as diffusivity depends on the temperature [10,19].
- -
At each deployment time, 2 samplers are removed from water and the sorbent placed inside the passive sampler is collected, deposited in a centrifuge glass vial or a polypropylene flask and extracted and analyzed generally using mass spectrometric methods coupled either to gas or liquid chromatography (GC–MS or LC–MS), according to the optimized methods for each specific chemical family [20].
- -
Evaluate compound stability as compounds degrading in water would not be accumulated in the CPS receiving phase. Each time a CPS is collected, an aliquot of water (1–5mL) is collected, taking care that the water volume does not decrease and guaranteeing that passive samplers are fully submerged. If LC–MS is used, water samples for the stability test can be analyzed direct sample injection. If GC–MS is used, water has to be extracted either with liquid-liquid extraction (LLE) or solid-phase extraction (SPE).
- -
Because the sampling rate can vary depending on the type of water to be monitored, it is suggested to calibrate the CPS first in bottled water to determine optimum performance and then with the water to be analyzed to refine the Rs and De values considering that organic matter, particulate material and other dissolved substances in water. These parameters will affect the diffusivity of compounds, and thus, their sorption in the receiving phase.
Finally, the concentration of contaminants in water (Cw) is determined based on the Rs and as a function of time (t) that the CPS has been deployed, according to Eq. (6).
where M is the amount (ng, μg) of compounds accumulated in the passive sampler. Once sampling rates with a specific passive sampler are calculated for a given contaminant in a given type of water, no further calibration is needed.
After calibration is performed under laboratory conditions, CPS are ready to be used in the field. A potential drawback that may arise is that the uptake of chemicals can be affected by external parameters, such as temperature, salinity, pH or water flow. These variations have to be studied and understood under controlled laboratory conditions before results can be meaningfully understood from passive samplers field deployment.
Properties and applicability of the ceramic passive samplersThe main characteristics of the customized CPS are:
- -
Effectiveness: deployment for a period of 1–4 weeks allows the determination of contaminants in surface water at trace levels (ng/L).
- -
Integrity and stability: they allow the identification of a large number of compounds present in a given area in a time-integrated approach, in a way that improve and/or complement the chemical information obtained with grab sampling.
- -
Capacity: high retention capacity of pollutants of different physico-chemical properties by optimizing the type and amount of sorbent(s) placed in the inner cavity of the CPS.
- -
Enrichment Factors: high preconcentration capacity even in clean waters with little contaminant loads.
- -
Versatility: allows the determination of pollutants in various types of water (river, waste, underground, marine, etc.) without loss of efficiency provided adequate laboratory-calibration has been performed.
- -
Compliance with regulations: systems capable of detecting legislated pollutants, complying the environmental quality standards imposed by European legislations.
- -
Autonomy: electricity or any other source of energy is not required.
- -
Durability and reusability: no loss of effectiveness in passive samplers deployed several times once sonicated and rinsed with acetone in between deployments.
- -
Cost: they are affordable systems, with a cost of € 25.
Among the different designs tested, we finally came up with three passive samplers where the morphology and density of the pore microstructure was optimized so that the rate of contaminant uptake was modulated to facilitate its deployment in different types of water and in different exposure periods [12]:
- -
Porous ceramic passive sampler (PCPS): samplers with medium porosity to be deployed for long periods of time, so as to have a monthly or yearly integrated concentration of contaminants in waters with low level of organic matter (drinking/groundwater/open seawater).
- -
Superporous ceramic passive sampler (SCPS): samplers with high porosity to be deployed for medium periods of time, so as to have a weekly integrated concentration of contaminants in waters (river water/coastal seawater).
- -
Macroporous ceramic passive sampler (MCPS): samplers with high porosity and small wall thickness for very rapid uptake of contaminants, being especially adequate to be deployed in short periods of time, so as to have 24h or weekly integrated concentration of contaminants in “dirty” waters without the risk of fouling or clogging (wastewaters).
The advantage of customizing the porosity of the CPS is that they can be deployed in different types of water and during various time periods to determine contaminants that can affect water quality. Their foreseen applications are:
- 1.
Monitoring of priority pollutants: the ceramic sampler allows monitoring the presence of priority or legislated pollutants in surface waters according to the environmental quality standards set by the European Directive 2013/39/EU on water policy.
- 2.
Monitoring of emerging unknown pollutants: the device allows the non-target screening of contaminants adsorbed in the inner sorbent and permits the identification of new compounds by using high resolution mass spectrometry based techniques.
- 3.
Control of industrial discharges: the device allows the identification of specific pollutants in effluents from automobile, paper, textile, tanning, food, plastics, dyes, etc. industries by placing the CPS in the discharge point.
- 4.
Control of pharmaceuticals at the source of pollution: placing the CPS in hospital effluents, in senior-residences effluents, pharmaceutical industries or aquaculture installations.
- 5.
Control pf pesticides (and other contaminants) in irrigation water canals or swamps: determination of hazardous substances that are harmful to people or crops.
- 6.
Control of effluents from WWTPs (wastewater treatment plants): the monitoring of recalcitrant pollutants (which are not eliminated during the biological treatment of WWTPs) in effluents allows improving treatment and adequately managing water discharge according to potential reuse.
- 7.
Control of the drinking water: the use of samplers at the outlet of the drinking water works or at the bottling plant allows the efficient monitoring of water quality.
The customized samplers offering different diffusivity and contaminant uptake can be deployed in different types of water and during different periods without the problem saturation. In practice, when deployed in real environmental waters, many passive samplers suffer from fouling and clogging and this decreases the uptake of contaminants, and thus, affects their performance. Fouling and clogging occurs when waters have a high organic content, when they are very eutrophic, or contain high amounts of suspended particulate matter. This is very common in Mediterranean rivers with little flows, in wastewaters or coastal seawaters, and this effect is amplified when passive samplers are deployed for long periods of time. Other problems that can arise is that CPS are stolen when deployed in areas with no control (harbors, rivers), and unfortunately no solution can be provided here.
Fig. 5 shows some examples where CPS have been implemented and their aspect upon deployment in different types of waters. Fig. 5A shows passive samplers installed for 10 days inside a harbor close to Barcelona to determine diethylphthalate (DEP), benzophenone (BP), bisphenol A (BPA), octyl- (OP) and nonylphenol (NP). Calibration under laboratory conditions provided a net accumulation of OP and NP from 45 to 1000ng after a deploying period of 10 d, that corresponds to a sampling rate of 0.03, 0.03, 0.03, 0.28 and 0.126Ld−1 for DEP, BP, BPA, OP and NP, respectively (Fig. 5A). After deployment in coastal seawaters, the only 2 compounds detected were OP and NP at absolute amount of 1300 and 250ng, corresponding to 467 and 198ngL−1, respectively. The other compounds were not detected due to the low sampling rates obtained. In Fig. 5B, passive samplers were installed at the inlet and the effluent of a wastewater treatment plant in Barcelona to determine anticancer drugs. While it was impossible to analyze the inlet samplers because they were fully covered with mud, dirt and slime, the samplers installed in the outlet waters were sufficiently clean to be successfully analyzed. Its deployment permitted to detect main anticancer drugs, namely cyclophosphamide (CYC) and mycophenolic acid (MPA) at concentrations between 19±3 and 136±28ngL−1, and good agreement was observed between passive samplers and conventional grab sampling and solid-phase extraction [21]. In Fig. 5C, the CPS was tested for lindane, a pesticide used in the decade of the’70 to treat crops, seeds, forestry products, and to treat livestock, pets and humans to eliminate lice and scabies. Lindane is the most acutely toxic hexachlorocyclohexane isomer affecting the central nervous and endocrine systems. In Spain there was a main production factory in Sabiñanigo and in Flix and for years this compounds was released to the Ebro river basin. After laboratory calibration giving a Rs of 0.054Ld−1 (Fig. 5C), lindane was monitored in the Ebro river close to the Flix factory. However, the samplers were stolen during deployment and this meant that it is better to avoid installation of the CPS in non-controlled and free-access sites. Finally, Fig. 5D shows the Llobregat river, a typical Mediterranean river flowing along a highly anthropogenic area and suffering from water scarcity. In this river, passive samplers were deployed for one month in a controlled water station to determine perfluorinated alkyl substances (PFASs). Fig. 5D indicates the chromatogram of the water samples where it was possible to determine PFBS, PFHxA, PFHpA, PFOA and PFOS at absolute concentrations ranging from 1.1 to 26.7ng (for 30 d deployment). This was a first trial to evaluate if PFASs would diffuse through the CPS and future studies are being carried out to calibrate the system. These examples illustrate the versatility of the designed CPS to monitor contaminants of different chemical families, fueling its use for the routine integrated water monitoring.
Sites where ceramic passive samplers have been deployed and aspect of the sampler upon deployment: (A) phthalates in a coastal harbor in front of a cement plant indicating the CPS laboratory calibration; (B) wastewater treatment plant indicating K, Rs and the concentration in water [21]; (C) Ebro river for the monitoring of lindane, where the CPS were stolen as upon collection the cord form a riverine harbor was found cut; (D) PFAS in a very anthropogenic river with high loads of particulate matter where the CPS were collected full of mud but still PFAS were detected as shown in the LC–MS/MS chromatogram.
Overall, ensuring the long-term monitoring of contaminants in different water bodies with an easy to implement and cost-effective ceramic sampler will promote the control of the quality of water and implement remediation actions in areas affected by environmental pollution.
ConclusionsThe work done with Prof. Orera on the design, fabrication, testing and finally deploying ceramic passive samplers to face multiple aspects of water monitoring has been one of the most endearing collaborations we have ever had. The simple question as “how to start” triggered discussions regarding the material to be used, the dimensions of the sampler, how to attain the desired porosity and connectivity among porous, how to calculate diffusivity considering the (inter)porous structure and how to evaluate the performance of the samplers. First ceramic samplers fabricated were too thick and small and were ineffective as the sampling rate was very low. Then, the wall thickness was reduced to overcome this problem, but then it was evidenced that the samplers became fragile and would break when capped or deployed in the environment. After several simulations and numerical optimization, it was found that the ceramic membrane porosity had a central role in modulating the diffusivity of contaminants, and specifically, the importance of the inter-connected porosity to allow a high sampling rate. With this respect, several porous structures were developed and tested using methylene blue as calibrant, in a way to evaluate the diffusivity, saturation and compare the performance of the different passive samplers developed. This information was crucial to define the applicability of different structures for monitoring different types of water and at varying periods of times, ranging from days to months. The research developed was scientifically challenging and personally motivating, as a step-by step procedure was required at each developmental stage. The outcome of this research was the full development of 3 ceramic structures that are operative for the integrated monitoring of contaminants in waters and have been deployed at experimental level and their efficiency demonstrated at TRL 6. Our final dream was to have a system capable to detect the load of contaminants in a simple and effective way so that it could be easily implemented to control main pollutants present in waters and identify main areas affected by chemical pollution so that contaminant mitigation strategies could be implemented. This was a very high ambition and aimed to promote ceramic passive samplers as a routine water monitoring strategy aimed at environment protection, to contribute to the “zero pollution” goal as proposed by the European Union for a safer and healthier environment. The knowledge, excellence, endeavor, motivation, enthusiasm and resolution is the legacy that Prof. Orera has left for those who still want to fight against chemical pollution.
This work has been financed by the Ministry of Science and Innovation of Spain (project PID2019-105732GB-C21) and by the project IMAQUA (COMRDI16-1-0063) of the RIS3CAT call financed by the Fons Europeu de Desenvolupament Regional de la Unió Europea in the frameof FEDER program of Catalunya 2014-2020. Marina Erb is acknowledged for analyzing phthalates and alkylphenols and Maria Dulsat for analyzing PFASs.