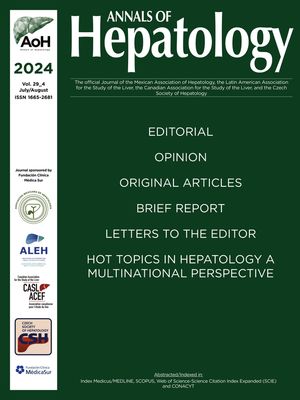
Edited by: Marco Arrese - Pontifical Catholic University of Chile, Santiago, Chile
More infoMetabolic Dysfunction-Associated Fatty Liver Disease and Diabetes Mellitus are two prevalent metabolic disorders that often coexist and synergistically contribute to the progression of each other. Several pathophysiological pathways are involved in the association, including insulin resistance, inflammation, and lipotoxicity, providing a foundation for understanding the complex interrelationships between these conditions. The presence of MASLD has a significant impact on diabetes risk and the development of microvascular and macrovascular complications, and diabetes significantly contributes to an increased risk of liver fibrosis progression in MASLD and the development of hepatocellular carcinoma. Moreover, both pathologies have a synergistic effect on cardiovascular events and mortality.
Therapeutic interventions targeting MASLD and diabetes are discussed, considering lifestyle modifications, pharmacological agents, and emerging treatment modalities. The review also addresses the challenges in managing these comorbidities, such as the need for personalized approaches and the potential impact on cardiovascular health. The insights gleaned from this analysis can inform clinicians, researchers, and policymakers in developing integrated strategies for preventing, diagnosing, and managing these metabolic disorders.
MASLD (metabolic dysfunction-associated steatotic liver disease) is characterized by the presence of liver macrosteatosis in >5% of the hepatocytes. Its prevalence increases in conditions involving insulin resistance, such as obesity, type 2 diabetes mellitus (T2DM), and metabolic syndrome. MASLD frequently presents as a mild disease with a low impact on liver function (75–80%), however in 20–25% of cases, it presents as metabolic dysfunction-associated steatohepatitis (MASH), which represents a fibro-inflammatory phenotype that can progress to liver cirrhosis and increase liver-related death [1].
MASLD and type 2 diabetes mellitus (T2DM) are currently in a global pandemic situation associated with high public health burdens and costs. The worldwide prevalence of T2DM was estimated to be 529 million individuals in 2021, affecting 10.5% of the adult population. Moreover, the prevalence is expected to increase to 1.23 billion by 2050 [2]. Unfortunately, a great proportion of T2DM subjects are undiagnosed, and more than 50% of diagnosed patients present a suboptimal metabolic control. For example, in Latin America, one-third of patients are undiagnosed, and 52–72% have unmet glycemic control [3,4].
Regarding MASLD, it currently represents one of the most common causes of liver disease in the globe and is a leading cause of liver-related mortality. In a recent meta-analysis of 72 observational studies from 17 countries, the prevalence of MASLD in adults increased from 255% in publications before 2005 to 378% in reports from 2016 or later [5]. Latin America has reported one of the highest MASLD prevalence worldwide (31%) [6,7]. MASLD is currently the liver disease with the highest increase in disabled-adjusted life years (DALYs) [8]. Early detection and appropriate management are mandatory to tackle this health and economic burden.
MASLD and T2DM cohabit in a bidirectional symbiotic relationship. T2DM is one of the most critical risk factors for MASLD. The estimated prevalence of MASLD in the diabetic population was 65%, based on a recent meta-analysis of 152 studies [9]. Notably, more aggressive forms of MASLD are also higher in the diabetic population, with an estimated prevalence of MASH at 31.6% and advanced fibrosis at 15% [9,10,11]. MASLD is an important risk factor for developing T2DM as well. This risk was recently reviewed in a meta-analysis of 33 studies estimating an HR=2.2 (95% CI 1.98–2.43), and the risk was increased by the presence of more advanced fibrosis (F2-F4) (HR=2.69) or steatosis (HR=2.4) [12].
The synergic relation is not limited to liver disease and T2DM incidence. Multiple extra-hepatic complications are also enhanced in subjects who are carriers of both diseases, such as cardiovascular disease (HR 1.45), chronic kidney disease (HR 1.43), and colorectal tumors (HR 3.08) [13,14] (Fig. 1). The pathogenic pathways involved in this frequent association will be explored in the next section.
2Pathogenic pathways involved in the synergic interaction2.1Insulin resistance, liver lipotoxicity, and adipocyte dysfunctionInsulin resistance is the main driver for MASLD and T2DM. Its presence is sine qua non for both diseases. In the liver, insulin resistance is mainly manifested by disrupted regulation of glucose metabolic pathways, such as increased neo-glucogenesis and glycogenolysis and decreased glycogen synthesis. As a direct consequence, hyperglycemia is developed, and pancreatic β cells respond by increasing insulin secretion to maintain glycemic control. The insulin response in skeletal muscle, one of the main organs involved in glucose consumption, is dysregulated, reducing glucose transport and glycogen synthesis in myocytes [15]. This metabolic derangement induces a chronic state of hyperinsulinemia that, in the long term, cannot sustain normoglycemia developing T2DM. Insulin resistance in peripheral adipose tissue increases lipolysis and free fatty acid production, which are avidly captured by hepatocytes. In the liver, lipid metabolic pathways such as sterol regulatory element-binding protein (SREBP) and carbohydrate regulatory element-binding protein (ChREBP) are enhanced by hyperinsulinemia and hyperglycemia respectively, inducing a dysregulated enhanced lipogenesis and liver steatosis. The hepatocyte responds to the increased lipid supply by incrementing the lipid export to systemic circulation as VLDL, which is responsible for the characteristic atherogenic dyslipidemia observed in both conditions.
With disease progression, lipids overflow surpasses the hepatocyte compensatory mechanisms such as lipid export and fatty acid oxidation. A significant amount ends up accumulating in lipid droplets in their cytoplasm. Several by-products of lipid metabolism have been described as accumulating in the hepatocyte droplets. Notably, diacylglycerol and ceramides are lipid products of significant importance since they have been consistently associated with inflammation, insulin resistance, hepatocyte dysfunction, endoplasmic reticulum stress, oxidative stress, inflammasome activation, and liver fibrosis, constituting a major pathway of synergy in the presence of T2DM and MASLD [16].
Adipose tissue dysfunction is another hallmark of insulin resistance and T2DM. Increased adipose tissue volume, particularly visceral adipose tissue, triggers adipocyte inflammation and increased cytokine production, such as TNFα, IL-1, IL-6, IL-12, and MCP1 [17]. Adipokine production is modified to a pathogenic signature with increased leptin, angiopoietin 2 (ANGPT2), chemerin, apelin, and visfatin, and decreased serum levels of adiponectin and omentin-1 that is associated with increased progression of liver disease, pancreatic β-cell loss, and cardiovascular complications [18–20].
2.2Microbiota dysbiosisMicrobiota is another important element in metabolic dysfunction. Intestinal flora (including bacteria, fungi, viruses, and protozoa) closely interact with intestinal cells through several receptors, including toll-like receptors (TLRs) and intranuclear-binding oligomerization domain-like receptors (or NOD-like receptors). Microorganisms products (e.g., lipopolysaccharides, DNA, peptidoglycans) penetrate through the gastrointestinal barrier and directly interact with liver cells, including hepatocytes, Kupffer cells, sinusoidal cells, and dendritic cells, among others. MASLD is characterized by several microbiota changes (dysbiosis) associated with increased intestinal permeability that promote MASLD progression. MASLD patients have lower proportions of Bacteroidetes and higher proportions of Prevotella and Porphyromonas spp[21]. This dysbiotic phenotype is also present in T2DM [22]. Ethanol-producing bacteria such as E. coli and Klebsiella are also increased in subjects with NASH, and this is associated with increased blood concentration of alcohol [23]. Other relevant pathogenic bacterial metabolites have been described to be involved in MASLD pathogenesis, such as trimethylamine N-oxide (TMAO) [24]. T2DM subjects present reduced Akkermansia muciniphila, Bacteroides acidifaciens, and Lactobacillus gasseri, which is associated with reduced fatty acid oxidation and energy expenditure in adipose tissue promoting MASLD pathogenesis [22]. In addition, case-control studies demonstrate that liver cirrhosis and T2DM share features of reduced Akkermansuia and Bifidobacterium and might be related to systemic inflammation patterns observed in the process of hepatocarcinogenesis [22,25,26]. Hence, microbiota dysbiosis can explain part of the increased risk of MASLD and hepatocellular carcinoma (HCC) in T2DM.
2.3Bile acid metabolic pathwaysActive research is being conducted on bile acid signaling as a major regulator pathway in glucose and lipid metabolism with potential implications in the treatment of diabetes and MASLD [27]. Two major bile acid receptors are involved in this pathway: farnesoid X receptor (FXR) and G-protein-coupled bile receptor 1 (GPBAR1), also known as Takeda G-protein coupled receptor 5 (TGR5). FXR cognate ligands are primary hydrophobic bile acids, mainly chenodeoxycholic acid (CDCA) and are expressed in enterocytes and hepatocytes. In the liver, FXR activation inhibits bile acid (BA) synthesis, and in enterocytes, FXR induces FGF19 secretion, which inhibits BA synthesis in the liver through the activation of the FGFR4/β-klotho complex. In addition, FXR activation in the liver reduces lipogenesis, increases fatty acid oxidation, reduces neo-glucogenesis, and induces glycogen synthesis, particularly in the fed state. FXR receptor is involved in the regulation of inflammatory and fibrotic response in the liver by a direct effect on stellate cells and NK cells [28]. TGR5 receptor ligands are secondary hydrophobic bile acids, mainly lithocholic acid and deoxycholic acid. TGR5 is expressed in enterocytes, muscles, immune organs, the enteric nervous system, and adipose tissue. TGR5 activation in ileal and colon L-cells induces secretion of Glucagon-like peptide 1 (GLP-1). GLP-1 actively promotes insulin secretion in β-cells and inhibits glucagon production in α-cells of the pancreas [28]. TGR5 also induces a central anorexigenic response in the hypothalamus and increases basal metabolism and energy consumption in brown adipose tissue and skeletal muscle by increasing the conversion of the inactive thyroxine (T4) to active 3,5,3-triiodothyronine (T3) [29,30]. BA metabolism modifications have been described in subjects with MASH, independent of the presence of obesity and T2DM. Most authors describe an increased primary to secondary BA and conjugated to unconjugated BA ratios. In bariatric surgery, particularly gastric bypass, there is an increased exposure of BA to the ileum, activating FXR and TGR5 receptors. This effect has been described as being involved in the positive metabolic effects of the intervention in T2DM, MASLD, and insulin resistance [31].
2.4Genetic factors for MASLD and T2DMGenetic predisposition has been extensively described for T2DM and MASLD. More than 400 distinct genomic regions have been report to influence T2DM risk. The genetic variants identified are reported to influence β-cell function (insulin production and release), insulin action (increased insulin resistance), and adiposity, among other functions [32]. Several genetic variants have been involved in predisposition to MASLD, most of them involved in hepatocyte lipid processing, including lipid droplet remodeling (PNPLA3, HSD17B13, MBOAT), synthesis and secretion of VLDL (TM6SF2), increased lipogenesis in response to glucose (GCRK). Abnormal lipid processing may increase lipotoxic mediators, which induce MASLD progression and increase hepatic insulin resistance and hyperglycemia [33,34].
3Clinical implications of the MASLD and T2DM association: surveillance, diagnosis, and stagingClinicians should be aware of the consequences of the symbiotic association between T2DM and MASLD. Due to the high prevalence and multisystemic implications, their management should not be restricted to liver specialists; on the contrary, it should be expanded to general practitioners, internal medicine, cardiologists, diabetologists, and endocrinologists, among other specialties. Table 1 describes the role of general physicians, diabetologists, and gastroenterologists/hepatologists in MASLD care in subjects with T2DM. The clinical implications of this relation will be detailed in the following sections and are summarized in Fig. 1.
Because of the 2-3-fold increased risk of diabetes incidence in MASLD patients, most guidelines recommend screening T2DM in MASLD patients. Active surveillance with serum fasting glucose and Hba1c should be included for MASLD patients. Since MASLD is a strong risk factor for T2DM, we recommend screening every 1-2 years, particularly in obese subjects or subjects with a family history of T2DM.
T2DM diagnosis has implications for liver fibrosis progression in MASLD and the risk of HCC. In a meta-analysis of 6 retrospective cohort studies (n=2016), with a median follow-up of 2.8 years, the presence of T2DM in MASLD patients increased the risk of hepatic decompensation (defined as the presence of ascites, encephalopathy, or variceal bleeding) with an adjusted HR=2.14 (95% CI=1.4–3.3). This result remained significant in the subgroup analysis of patients without cirrhosis (HR=2.5, P=0.03). In subjects with MASLD cirrhosis, T2DM represents a significant risk factor for HCC, with an HR=5.25 (95% CI=1.1–24.7) [35]. The presence of T2DM in subjects with MASLD should make clinicians aware of the risk of cirrhosis and its complications, including HCC in the cirrhotic subgroup.
Glycemic control of T2DM could play a protective role in MASLD progression. Some observational studies describe an association between higher Hba1c levels (>7%) and more advanced MASLD [36]. A recent meta-analysis demonstrated that increased Hba1c was associated with hepatic decompensation (HR=1.3; 95% CI=1.1–1.6) [35]. Future studies are needed to clarify this point. However, the benefit of metabolic control is pivotal for diabetes care and may impact MASLD progression [37].
3.2MASLD and microvascular complication of T2DMThe presence of MASLD is associated with an increased risk of microvascular complications of T2DM, in particular, renal failure and diabetic neuropathy. Regarding chronic kidney disease (CKD), a recent meta-analysis of 13 observational studies with a median follow-up of 9.7 years estimated an increased risk of CKD defined as glomerular filtration rate <60 mL/min/1.73 m2 in subjects with MASLD (HR=1.43; 95% CI 1.33–1.54) [38]. The risk of CKD is likely to be increased in advanced live fibrosis with HR 2.8–3.3 in subjects with histology confirmed ≥F3 [38,39]. In a study of 157 subjects with T2DM, I148M homozygosity of the PNPLA3 gene was independently associated with an increased risk of CKD (adjusted-OR 6.65; 95% CI 1.7–26.8, P<0.01) [40].
There is also evidence about the association between MASLD and diabetic neuropathy [41]. A recent prospective, case-control study (case n=1208; control n=1908) with a median follow-up of 5 years demonstrated an increased risk of neuropathy in MASLD subjects with an OR: 1.34 (95% CI: 1.1–1.6) [42]. The association between MASLD and retinopathy lacks clarity. A recent meta-analysis concluded no significant association between these entities (OR = 0.92; 95% CI: 0.7–1.3) [43].
3.3Cardiovascular disease in subjects with T2DM and MASLDCardiovascular disease (CVD) is the leading cause of death in T2DM and MASLD [44]. T2DM is associated with a 2-fold increase in coronary artery disease, cerebrovascular disease, heart failure, peripheral artery disease, and cardiovascular deaths [45]. Current studies have depicted a significant heterogeneity in the effect of T2DM on CVD risk. T2DM tends to cluster with additional risk factors, like hypertension, dyslipidemia, and obesity. More than half of T2DM patients present two of these risk factors, and 21 % present all three [46]. The association of these comorbidities and other essential factors such as age, sex, smoking, and ethnicity have been described to modulate the risk of CVD in both diseases.
MASLD should be considered a relevant CVD risk factor. A recent metanalysis of 36 observational studies involving 5.8 million people with an incident of approximately 100.000 cases of CVD events over a median follow-up of 6.5 years has demonstrated that MASLD is independently associated with increased fatal (HR: 1.3; 95% CI 1.1–1.6) and non-fatal CVD (HR: 1.4; 95% CI 1.2–1.6). CVD risk increases with the severity of fibrosis (HR: 2.5; 95% CI 1.7–3.7), and this association remained significant in the subgroup analysis of studies with non-invasive and histologic assessment. MASLD and T2DM have also been associated with cardiac remodeling and an increased risk of arrhythmias and heart failure [47–49]. Diagnosis and appropriate management of associated risk factors in subjects with combined T2DM and MASLD are mandatory to prevent CVD [50].
3.4Surveillance of MASLD and liver complications in patients with T2DMThe liver is the most frequent target organ comprised in T2DM, with an estimated prevalence of 65%, surpassing nephropathy (34%), retinopathy (35.4%), neuropathy (30%), and cardiovascular disease (47%). MASLD assessment on the T2DM population is currently recommended in most published guidelines. American Diabetes Association (ADA) has recently included MASLD screening in the diabetic population in their 2023 update of guidelines [51]. Relying on liver function tests to assess MASLD in diabetes is insufficient [52,53]. Often, subjects with advanced fibrosis only present with mild transaminase abnormalities. In a recent multicentric French study, 360 T2DM patients with ALT above 30 IU/L for women and 20 IU/L for men underwent liver biopsy for MASLD assessment. Although one-third of patients presented ALT 20–40 IU/L, they reported a prevalence of 58% MASH, 38% of advanced fibrosis, and 10% of cirrhosis [54]. This study emphasizes the importance of considering these cut-off values abnormal in subjects with T2DM.
Based on current guidelines, we propose a liver check-up in T2DM (Fig. 2). The first step is MASLD diagnosis. According to the new diagnostic consensus for MASLD, diagnosis is based on three criteria: (1) presence of liver steatosis; (2) presence of 1 of 5 cardiometabolic criteria (body mass index ≥25 gk/m2 or increased waist circumference; diabetes; hypertension, hypertriglyceridemia, dyslipidemia); (3) discard other causes of steatosis [55]. Because of its simplicity and availability, abdominal ultrasound (US) is the preferred method for steatosis detection. US can detect moderate liver steatosis with 84.8% sensitivity and 93.6% specificity, but this detection rate is reduced in mild steatosis (<30%) [56,57]. The accuracy of the US depends on the experience of the operator, the type of ultrasound device used, and patient characteristics, particularly obesity. There is also substantial interobserver variability in the diagnosis of steatosis (∼20%) [58]. In addition, US can also provide information about elements that suggest the presence of cirrhosis, such as splenomegaly, increased left lobe size, nodular liver surface, ascites, or the presence of portosystemic shunts.
Liver check-up of MASLD in subjects with type 2 diabetes (created with BioRender.com) GLP-1 RA, GLP-1 receptor agonist; FIB-4, Fibrosis-4; T2DM, type 2 diabetes mellitus; AST, aspartate aminotransferase, ALT, alanine aminotransferase; PCP, primary care physician; VCTE, vibration-controlled transient elastography.
Other image modalities for steatosis assessment include magnet resonance imaging (MRI) using proton density fat fraction (PDFF) and the ultrasound-based controlled attenuation parameter (CAP), which is integrated into the FibroScan® (echosens). These methods, particularly MRI-PDFF, are more precise for liver steatosis quantification. However, because of their cost and lower availability, they are not recommended for routine MASLD diagnosis. Of note, in MASLD with advanced fibrosis (F3-4), liver steatosis significantly decreases and almost disappears in cirrhotic patients, a phenomenon described as burn-out MASLD [59]. Hence, in cirrhotic patients, the absence of steatosis does not exclude MASLD as ethiology.
The second step of liver check-up in T2DM is to discard other causes of steatosis such as alcohol, medications (e.g., methotrexate, amiodarone, glucocorticoids, tamoxifen, valproate), and chronic viral hepatitis C. Occult alcohol consumption in MASLD patient is frequent. A recent study demonstrated that using ethyl glucuronide in hair and urine as a biomarker detected moderate to severe occult alcohol consumption in 25% of subjects who were previously diagnosed as MASLD [60]. Since alcohol consumption significantly increases the risk of cirrhosis and liver complications in MASLD, a new category for subjects with combined MASLD and increased alcohol intake (MetALD) was created to characterize this population further. Active alcohol use disorder screening is recommended, and appropriate management, including a multidisciplinary approach, should be considered in patients with dual etiology [61]. In patients with sustained elevated liver transaminases or transaminases elevated more than 10 times the upper normal limit, an evaluation by a gastroenterologist/hepatologist to rule out further other causes of liver disease such as autoimmune hepatitis, hemochromatosis, Wilson disease, alpha-1 antitrypsin, celiac disease and monogenic disease with liver steatosis.
The third step (probably one of the most important) is assessing MASLD severity. Since liver fibrosis is the main prognostic factor of liver-related complications and death, severity assessment is focused on grading liver fibrosis [62]. Non-invasive tests (NITs) for fibrosis can be classified into three types: (1) simple serum NITs, (2) specific serum NITs, and (3) imaging-based NITs. Several simple serum NITs have been used for MASLD (Fib-4, NAFLD fibrosis score, APRI, AST to ALT ratio). The most recommended method is FIB-4, which is based on standard laboratory tests (age, aspartate aminotransferase, alanine aminotransferase, and platelet count). FIB-4 categorizes patients according to their risk of presenting advanced fibrosis (F3-4) into three categories: (1) low-risk FIB-4<1.3; (2) indeterminate risk FIB-4 1.3-2.67; (3) high-risk FIB-4 >2.67 (Fig. 2). The advantage of using this test is its availability, simplicity, and high negative predictive value for advanced fibrosis (90%) [63]. Although the accuracy of FIB-4 is mildly reduced in T2DM [64], it is still the most recommended first approach NIT in most guidelines and has been demonstrated to be cost-effective [65–67].
Subjects with FIB-4 scores in an indeterminate or high-risk category should be assessed with a second NIT to clarify their fibrosis assessment further. For this purpose, we can use a specific serum NIT or an imaging-based NIT. Specific serum NITs are based on the measurement of biomarkers of liver fibrosis that are not part of routine laboratory assays. Some are based on a single biomarker (e.g., ProC3, WFA þ-M2BP) or a composite set of biomarkers such as ELF score, Fibrotest, Fibrometer, and Hepascore. The AUROC of specific NITs ranges between 0.73–0.94 and is better for composite NITs, particularly Fibrotest and Fibrometer [63]. These tests are useful in scenarios where there are access limitations for image-based NITs [65].
The preferred method for further assessment is imaging-based NITs. Most of them are based on assessing liver stiffness by different methods correlating to the degree of liver fibrosis. However, it is important to consider that other factors can increase liver stiffness such as cardiac failure, inflammation, steatosis, post-prandial state, and liver congestion. US-based elastography is the most recommended NIT for assessing liver fibrosis after simple serum NITs. The most used methods are VCTE (vibration-controlled transient elastography or fibroscan®), point shear wave elastography, and 2D-SWE (2-dimensional shear-wave elastography). VCTE and 2D-SWE are more precise with AUROC 0.8–0.9 for detecting F3-4 and both demonstrated comparable results in recent head-to-head analysis [68,69]. MRI-based NIT is more accurate than US-based [70] and combined with the FIB-4 score, has excellent prediction capacity for liver-related events (liver decompensation, HCC, or death) with a negative predictive value of 99% at five years [71]. Although MRE-based elastography is more expensive and less available, it is a good option for patients who cannot be appropriately assessed by US elastography or have discordant results between their NITs assessment.
Liver biopsy remains the gold standard for MASLD fibrosis assessment. However, it has important limitations, such as its invasive nature with risk of complications and significant inter-observer variability of the assessment. Current indications have been limited to subjects who cannot be appropriately assessed their risk by NITs (e.g., NITs discordance), subjects with a possible alternative or concomitant diagnosis for another liver disease (e.g., autoimmune hepatitis, hemochromatosis, Wilson disease, etc.), clinical trials that use histologic scores of activity of MASLD, and subjects with persistently elevated liver blood tests despite proper treatment adherence.
We highlight the role of General Physicians, diabetologists, and gastroenterologists/hepatologists in liver care of T2DM. All of them should conduct MASLD diagnosis, risk stratification, and treatment in T2DM. Subjects with intermediate and high risk should be assessed by a gastroenterologist/hepatologist. Subjects with significant fibrosis should receive intensive treatment to prevent the development of cirrhosis. Subjects who are already cirrhotic should be screened to detect clinically significant portal hypertension and start beta-blocker therapy to prevent cirrhosis decompensation and variceal bleeding. HCC surveillance with abdominal US and alpha-fetoprotein every six months should also be started to detect and treat early lesions with curative treatments. Additional treatment for cirrhosis includes education, avoiding hepatotoxic agents, preventive immunizations, appropriate nutrition, treatment of sarcopenia and bone disease, and treatment of ascites and encephalopathy. Subjects with decompensated cirrhosis should be referred to liver transplantation centers.
4Therapeutic interventions for T2DM and MASLD4.1Lifestyle interventionsLifestyle intervention is the mainstay treatment for T2DM and MASLD. Obesity is one of the major drivers of the pathogenesis of both diseases. Hence, weight loss is the main aim of overweight and obese patients. A weight reduction of >7% is associated with MASH resolution in 64%, and >10% decreased weight is associated with MASH resolution in 90% and liver fibrosis regression in 81% of subjects [72]. Weight loss induces a better metabolic control of T2DM. Regarding macronutrient composition, carbohydrate restriction is needed for metabolic control in diabetes and can help to reduce body weight. Mediterranean diet is the preferred recommendation because of solid evidence on the reduction of diseases associated with MASLD and T2DM, such as cardiovascular events, stroke, cardiovascular mortality, and all-cause mortality [73,74]. Evidence on other types of diet, such as intermittent fasting or ketogenic diet, is based only on short-term studies with variable results; they are recommended in specific patients supervised by dietitians.
Exercise is also recommended to complement nutritional intervention because It has demonstrated an additive effect on liver function tests and insulin resistance [75]. Exercise in T2DM contributes to achieving glycemic control [76]. Aerobic or resistance exercise has comparable benefits and moderate to vigorous exercise >150 min/week is recommended [77]. Additional lifestyle recommendations include avoiding alcohol consumption in ≥F2 fibrosis, increasing coffee intake, keeping healthy sleeping habits, and smoking cessation.
4.2Cardiovascular disease preventionTo prevent cardiovascular events is a major significant target for treating subjects with T2DM. Controlling the multiple CVD risk factors associated with T2DM and MASLD (blood pressure, Hba1c, blood cholesterol, smoking) is of significant importance since it can reduce CV events by approximately 62% [50]. Aggressive treatment of hypertension and dyslipidemia is recommended. Hypertension medication should target blood pressure <130/80 mmHg; however, this target should be adapted individually and a higher target should be considered in elderly or fragile patients [44]. Angiotensin receptor antagonists and angiotensin-converting enzyme inhibitors are first-line therapies in diabetic patients with albuminuria; other options include thiazide diuretics and calcium channel blockers.
Management of blood cholesterol should be customized according to the patient's estimated CV risk. Statins are first-line therapy and are safe to use in subjects with MASLD. US guidelines recommend that all subjects with T2DM between 40 and 75 years old should be considered to start statin therapy at moderate intensity (LDL reduction of 30–50%) [78]. Clinical guidelines of the American Associations of Endocrinology and Hepatology (AACE and AASLD) recommend that subjects with T2DM and MASLD without other CV risk factors should target LDL <100 mg/dL [67]. High-intensity statin therapy and ezetimibe aimed to reduce LDL cholesterol by ≥ 50% should be started in subjects with multiple risk factors or 10-year estimated risk estimated ≥20% assessed by ASCVD risk estimator plus (https://tools.acc.org/ASCVD-Risk-Estimator-Plus/#!/calculate/estimate/). Regarding triglycerides, subjects with moderate hypertriglyceridemia (175–500 mg/d) should address and treat lifestyle factors (obesity and metabolic syndrome), secondary factors (diabetes mellitus, chronic liver or kidney disease and/or nephrotic syndrome, hypothyroidism), and medications that increase triglycerides. If hypertriglyceridemia persists after this initial management, starting statin therapy could be considered. In subjects with persistent severe hypertriglyceridemia (≥500 mg/dL), a very low-fat diet, avoidance of refined carbohydrates and alcohol, consumption of omega-3 fatty acids, and fibrate therapy should be considered [67,78].
Primary CVD prevention with antiplatelet agents can be of potential benefit in a subgroup of T2DM patients. The ASCEND trial (n=15,480 patients) randomized subjects with diabetes but no overt CVD to aspirin 100 mg daily or placebo. At a mean follow-up of 7.4 years, there was a 1% absolute reduction in major cardiac and cerebrovascular events among these low-risk patients treated with aspirin (8.5% vs. 9.6%, P=0.01) but a 1 %increase in major bleeding events (4.1% vs. 3.2%, P=0.003) in that same group. Bleeding events were primarily gastrointestinal and other noncranial origin, with no excess in fatal bleeding [79]. A second trial (ASPREE) demonstrated that aspirin did not reduce CV events in subjects ≥70 years old (≥65 in Afro-Americans and Hispanics) but significantly increased bleeding events [80]. The benefit of antiplatelet therapy for primary prevention is restricted to subjects with T2DM aged 50–70 years old and increased CVD risk with low risk of bleeding and should be considered individually [44].
4.3Bariatric surgeryIn patients with obesity and T2DM or high-risk MASLD, bariatric surgery can induce sustained, major weight loss. The metabolic effects of this type of surgery significantly improve glycemic control in T2DM patients [81]. The long-term effects of bariatric surgery in MASLD were described in a prospective study open-label French study of subjects with MASLD undergoing bariatric surgery with follow-up biopsies at 1 and 5 years. At 5-year MASH was resolved in 84%, fibrosis decreased in 70% of patients. Fibrosis reduction is progressive, beginning during the first year and continuing through 5 years [82]. A recent randomized controlled trial was conducted in 431 subjects with biopsy-proven MASH. They were randomized 1:1:1 to lifestyle modification plus best medical care, Roux-en-Y gastric bypass, or sleeve gastrectomy and followed for one year. The probability of MASH resolution was 3.6 times greater (95% CI 2·19–5·92; p<0·0001) in the Roux-en-Y gastric bypass group and 3.7 times greater (2·23–6·02; p<0·0001) in the sleeve gastrectomy group compared with in the lifestyle modification group [83]. These data strongly support the beneficial effects of bariatric-metabolic surgery in subjects with T2DM and MASLD.
Subjects with body mass index ≥ 40 kg/m2 or ≥ 35 kg/m2 with comorbidities (T2DM or pre-DM, uncontrolled hypertension, osteoarthritis of hip or knee, urinary incontinence) with inappropriate response lifestyle and medical therapy are considered candidates for bariatric surgery [84]. MASH, particularly with liver fibrosis, is currently also considered a comorbid condition for this indication [65]. However, a careful selection and preoperative preparation of candidates is crucial. Post-operative complications can eventually be severe and require hospitalization and reoperations. In addition, late weight regain has been described in up to 20% of patients, usually related to progressive non-compliant eaters [85]. Multidisciplinary care before and after surgery is relevant to prevent and treat these complications and provide nutritional and psychological support. The presence of liver cirrhosis is a setting where the benefits of this surgery are less clear. It can be conducted in selected patients with compensated disease in highly specialized bariatric units. The presence of decompensated cirrhosis or clinically significant portal hipertensión is associated with significantly increased post-operative mortality and should be considered a contraindication for this intervention [86].
4.3Pharmacologic treatment for MASLDAt present, no medication for MASLD has been recommended specifically for MASLD therapy, although some medications have been studied in randomized controlled trials. In a three-arm RCT that compared Vitamine E, pioglitazone, and placebo, vitamin E (800 UI per day for 96 weeks) was demonstrated to improve histology (reduction of NAS>2 points) compared to placebo in the PIVENS trial. This study excluded patients with diabetes, and there was no effect on liver fibrosis [87]. There are no prospective long-term studies with vitamin E and there is some concern about adverse effects, including increased risk of hemorrhagic stroke and prostate cancer. There is scarce evidence to support its use in MASLD and T2DM populations. Several new drugs are in development; some of them are currently studied in phase 3 trials: resmetirom (an agonist of thyroid receptor β), semaglutide (GLP-1 agonist) and lanifibranor (pan PPAR agonist). An updated review of the topic was recently published by Arnold et al. [88].
4.4Pharmacologic treatment for T2DM in patients with MASLDPatients with T2DM can benefit from medications that can potentially have positive effects on MASLD and liver disease. Metformin is the first-line pharmacologic therapy for T2DM; however, a direct effect on MASLD histology has not been demonstrated [89]. Metformin can be safely used in compensated cirrhosis and can potentially reduce HCC incidence [90]. Pioglitazone has been demonstrated to increase MASH resolution (OR=3.22; 95% CI 2.17–4.79) and reduce fibrosis progression (OR=1.66; 95% CI 1.12–2.47) in subjects with MASLD and T2DM or prediabetes [91]. Glucagon-like peptide-1 receptor agonist (GLP-1RA) has beneficial effects like weight loss and cardiovascular risk reduction in diabetic patients [92]. In addition, two RCTs have demonstrated significant effects of GLP-1RA in histologic outcomes of MASLD. A preliminary small study with liraglutide (1.8 mg/d for 48 weeks) in a small RCT of 52 patients was demonstrated to significantly increase MASH resolution (39 % vs. 9 %, P=0.02) and reduce fibrosis progression (9% vs. 36%, P=0.04) compared to placebo [93]. A second phase 2 RCT was conducted with semaglutide in daily dose for 72 weeks in subjects with biopsy-proven MASH. Resolution of steatohepatitis was achieved in 59% at the higher dose (equivalent to 2.4 mg/week semaglutide) compared with 17% in the placebo group (P < 0.001). No significant reduction of fibrosis was observed. However, fibrosis progression was significantly reduced (59% vs. 17%, P<0.001) for the high-dose group [94]. Currently, there is an ongoing phase 3 trial for 5-year therapy with semaglutide in subjects with MASLD and F2-3 stage of fibrosis. Based on this data, current ADA guidelines published in 2023 have recommended GLP-1 RA or pioglitazone as the preferred agents for the treatment of hyperglycemia in adults with T2DM biopsy-proven nonalcoholic steatohepatitis, or those at high risk for MASLD with clinically significant liver fibrosis using non-invasive tests [51].
Other agents have demonstrated promising results in MASLD, like tirzepatide (a dual glucose-dependent insulinotropic polypeptide and glucagon-like peptide-1 receptor agonist) and sodium-glucose cotransporter inhibitors. Both of them induce weight loss and liver steatosis reduction [95,96]. Nevertheless, further studies, including histologic assessment need to be conducted to further recommend these treatments in MASLD.
4ConclusionsThis review illuminates the intricate relationship between T2DM and MASLD, detailing their shared pathophysiological mechanisms and the mutual impact on each other's progression. The coexistence of these conditions poses significant challenges in patient management, emphasizing the need for integrated approaches.
The intricate interplay of insulin resistance, inflammation, and hepatic lipid metabolism serves as a key nexus linking T2DM and MASLD. Lifestyle modifications, including weight management and physical activity, emerge as central pillars in the prevention and management of both conditions. GLP-1 RA and pioglitazone therapy have been included in recent guidelines for the treatment of T2DM subjects with MASLD. Aggressive CVD risk factors management is necessary to reduce mortality. Furthermore, advancements in pharmacological interventions hold promise in addressing the complex metabolic pathways involved.
As research continues to unveil the molecular intricacies of T2DM and MASLD, therapeutic strategies need to evolve, focusing on personalized approaches that consider the overlapping components of these diseases and their extra-hepatic complications. A holistic understanding of the connections between T2DM and MASLD is pivotal for devising effective interventions that improve patient outcomes and reduce the global burden of these metabolic disorders.
FundingThe Chilean government partially supported this article through the Fondo Nacional de Desarrollo Científico y Tecnológico (FONDECYT 1211129 to DC).
Author contributionsFB, JU and MRG conceptualized the article's structure and designed figures. FB drafted the first MS. All the authors made substantial contributions to the conceptualization or design of the article and revised it critically for important intellectual content. All of the authors approved the final version that was submitted.