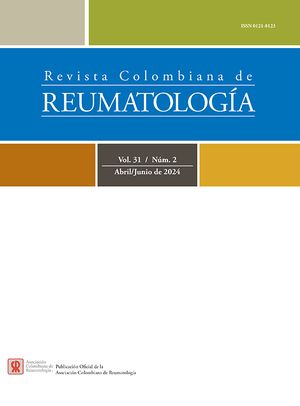
The presence of thrombotic events in COVID-19 patients has been described since the beginning of the pandemic. This association has been confirmed in most of the reported studies. Autopsy reports have shown that most thromboses are located in the lung, although they have also been observed in other organs such as the skin and kidneys. SARS-CoV2 infection induces a generalized prothrombotic state, which is attributed to a combination of factors such as hypoxia, excess cellular apoptosis, and mainly to overactivation of the immune system. Among immune-mediated prothrombotic situations, antiphospholipid syndrome (APS) stands out. Recurrent thrombotic events are observed in APS in the presence of antiphospholipid antibodies (aPL). There are numerous studies that report high prevalence of aPL in patients with COVID-19 infection. However, the results show discrepancies in the data on the prevalence of aPL, and its role in the pathogenesis of thrombosis in these patients. This could be due to the heterogeneity of the detection procedures for aPL or to transient elevations of non-pathogenic aPL levels in the context of infection. In this review we try to clarify the role of aPL in COVID-19 infection, and attempt to answer the question of whether it is a coagulopathy of its own, or secondary to APS.
La presencia de eventos trombóticos en los pacientes con COVID-19 se describió desde el inicio de la pandemia, asociación que ha sido confirmada en la mayoría de los estudios reportados. Los informes de necropsias han puesto de manifiesto que la mayoría de las trombosis se localiza en el pulmón, aunque también se han observado en otros órganos, como la piel y los riñones. La infección por SARS-CoV-2 induce un estado protrombótico generalizado que se atribuye a una conjunción de factores como la hipoxia, el exceso de apoptosis celular y, sobre todo, una hiperactivación del sistema inmune. Entre las situaciones protrombóticas inmunomediadas destaca el síndrome antifosfolipídico, en el cual se observan eventos trombóticos de repetición en presencia de anticuerpos antifosfolipídicos (AAF). Existen numerosos estudios que reportan una elevada prevalencia de AAF en los pacientes con infección por la COVID-19; sin embargo, los resultados muestran discordancias en los datos de prevalencia de AAF y su rol en la patogenia sobre la trombosis en estos pacientes, lo que que podría deberse a la heterogeneidad de los procedimientos de detección de los AAF o a elevaciones transitorias de los niveles de AAF no patogénicos en el contexto de la infección. En esta revisión se busca aclarar el papel de los AAF en la infección por COVID-19, intentando responder a la pregunta de si se trata de una coagulopatía propia o es secundaria a un síndrome antifosfolipídico.
Coronavirus disease-19 (COVID-19) is caused by the SARS-CoV2 virus and it is characterized by a heterogeneous and unpredictable course. In addition to asymptomatic forms, three stages of increasing severity have been identified in COVID-19.1 Most patients have the mildest form characterized by an acute infection, often olygosymptomatic or with flu-like symptoms.2 Around 15% of patients develop severe manifestations, including unilateral or bilateral pneumonia with progressive hypoxemia that cause acute respiratory distress syndrome (ARDS) requiring the use of mechanical ventilatory assistance. In the most serious form, there is a process of systemic hyperinflammation, with multi-organ involvement (cytokine storm), lymphopenia, and a strong elevation of acute phase reactants such as C-reactive protein, ferritin, D-dimers, cytokines, and chemokines with high risk of mortality.3 Data on histopathological samples on the various forms of COVID-19 that are based on autopsies of deceased patients are scarce. Microthrombi, diffuse alveolar damage, multiorgan thrombosis, hemophagocytosis, and immune cell depletion have been described in these autopsies.4
Overactivation of the innate immune system is very common in severe forms of COVID-19 and, surprisingly, lymphopenia is one of the usual findings in these patients, something that would be contradictory. This great activity of innate immunity triggers a state of hyperinflammation leading to a syndrome of massive cytokine release, characterized by ARDS, multiorgan dysfunctions and the presence of generalized microthrombi in capillaries and small vessels,5 a situation that resembles a thrombotic microangiopathy (TMA),6 a disseminated intravascular coagulation (DIC)7 or a catastrophic antiphospholipid syndrome (CAPS).8
General response to a new viral infectionWhen a virus infection occurs without prior contact with the immune system, a coordinated response of innate immunity and adaptive immunity is developed.
In the one hand, the innate immunity response has more rapid onset, it acts as the vanguard to initiate the control of the infection. It is carried out by the participation of a group of cells (neutrophils, monocytes and antigen presenting) and molecules effectors as the complement. These responses are characterized to be quick, stereotyped, and generally nonspecific. In the other hand, adaptive immunity is composed of cells and molecules that are specific antigens, and their effector capacity although it is more intense and prolonged than that of innate immunity requires a latency time. It can get started after recognition of the microorganism as foreign and the creation and subsequent elaboration of specific cells and molecules in sufficient quantity to carry out the control of the infection (primary response).9
In viral infections, as in other infections, the coordinated activity of innate and adaptive immunity is essential to generate an effective response.10 The mission of innate immunity is to try to resolve the infection quickly, and if not possible, contain it for as long as possible, necessary to develop an effective adaptive immunity response.
Although these responses in combination are effective in most infections, there are microorganisms that have the ability to escape the control of the immune system. These mechanisms include the incoordination of adaptive immunity generating insufficient and/or uncontrolled responses.11
Due to the ineffectiveness of the adaptive response, in a desperate attempt to control the infection, innate immunity contributes to the massive release of cytokines and the overactivation of local immune cells.12
SARS-CoV-2 infection produces a dysregulation with hyperactivation of adaptive immunity that leads to its exhaustion, which can be evidenced in total lymphocytopenia of both T and NK cells. This lymphopenia is directly proportional to the clinical severity.
After the control of adaptive immunity disappears, an overactivity of innate immunity occurs, leading to the massive release of cytokines of innate immunity (IL-1, IL-6, TNF-alpha), causing tissue damage and a hyperinflammatory state. This massive inflammatory response is not only ineffective but also leads to a situation of self-reactivity with the generation of a prothrombotic state. The sum of the damage generated by all these factors has fatal consequences at the systemic level.13–15
The baseline situation of the host before infection such as the elderly patients, presence of comorbidities, inherited situations of immunodeficiency, or drug treatments, add to the damage generated by the virus and the aberrant responses to infection. The combination of personal and infectious factors favor that the situation can become irreversible.16
Prothrombotic microenvironment in COVID-19Since the beginning of the pandemic, studies conducted in China described a large proportion of patients with elevated parameters associated with hypercoagulability, such as D-dimer, as well as a high incidence of thrombotic events in COVID-19 patients7,17,18 especially in those patients with more impaired lung function.19 Subsequently, reports emerged from research groups around the world that confirmed this higher incidence of thrombotic events, especially in patients at Intensive Care Units (ICU), where an incidence of 49% was described in patients with admissions greater than 2 weeks.18
Most of the thromboses (87%) were located in the lungs, this suggests a thrombosis secondary to local inflammation due to the virus.18 In many cases, these thrombotic events occurred even in patients receiving standard dose thromboprophylaxis. Laboratory tests revealed that patients with thrombotic events were those with elevated D-dimer, lactate dehydrogenase (LDH), fibrin and fibrinogen degeneration products, C-reactive protein, and moderate thrombocytopenia.20
However, many confounding factors coexist, for example the simple fact of admission to the ICU is a risk factor for thrombosis by itself.21 Furthermore, the presence of other known risk factors for thrombosis coincided in these patients: high age, smoking, and the presence of comorbidities such as high blood pressure, diabetes mellitus, and metabolic syndrome.22,23 Infection per se would be an additional coadjuvant factor for thrombosis, in addition, the conjunction of all these risk factors would lead to a highly prothrombotic situation.
Hypoxia and complementCoronavirus infections have been described as a trigger for thrombosis through several direct mechanisms: endothelial damage with elevated levels of von Willebrand factor; systemic inflammation, by activation of the Toll-like receptor; and activation of the tissue factor pathway.24 Additionally, the SARS-CoV2 infection can also activate thrombosis by indirect mechanisms, (1) The inflammatory response caused by cytokine storms can lead to complement activation that can lead to an immune-mediated hypercoagulability situation25 and (2) the alveolar lesions caused by the infection prevent a correct diffusion of gases that cause tissue hypoxia. It is well known that hypoxia induces a hypercoagulable situation that acts as a trigger for thrombosis.26
Hypoxia-associated thrombosis in COVID-19 patients has been observed in the elderly with comorbidities.27 Although it has also been observed in young patients with hypoxia without other risk factors for the development of thrombosis.28
Hyperinflammation secondary to increased apoptosisAnother factor that could indirectly participate in the prothrombotic situation is the large number of apoptotic cells that are generated as a consequence of infection or sepsis.29 The presence of microparticles and cellular debris from apoptotic cells has been described as promoters of thrombus triggering.30
The study of this phenomenon in COVID-19 can help us understand the pathophysiology of this disease. Recently it has been described that caspase-8, in addition to its apoptotic function, induces the expression of pro-inflammatory cytokines and cleave pro-IL-1β and IL-18, resulting in the release of bioactive cytokines and causing necroptosis.31 This is an immune-mediated cell death pathway. Its function is to kill virus-infected cells and activate immune (innate and adaptive) to decrease virus replication.32 However, the dysregulation of this mechanism can produce a potentially harmful local hyperinflammatory situation for uninfected cells (generalized pulmonary involvement) and also a systemic hyperinflammatory response as occurs in influenza A virus infection.33
The apoptosis and inflammation mediated by caspase-8 sustained over time in SARS-CoV2 infection may be eliciting an excessive immune response in lung tissue that is evidenced by the necrotic cell debris and diffuse alveolar damage seen in the lungs from autopsies of COVID-19 patients.34
The inflammatory process itself plus the formation of hyaline membrane in alveolar wall interfere with the correct gas exchange, which may cause local hypoxemia and tissue ischemia. This situation implies itself an increased risk of thrombosis.35
Hypoxia causes transcriptional changes in cells which proceed to elaborate hypoxia-inducible transcription factors (HIF-1 and HIF-2). These factors induce a decrease in protein S (anti-thrombotic) levels leading to an increase in thrombin levels36 as well as the expression of coagulant factors and integrins that stimulate thrombus formation through the neutrophils extracellular traps (NETs) pathway.37
The NETs are three-dimensional extracellular networks of decondensed chromatin that produces cell death called NETosis mediated by neutrophils. NETs are decorated with histones and antimicrobial proteins to trap and kill microbes as part of the innate immune system.38 The entrapment of microorganisms in DNA fibers prevents their spread and facilitates the concentration of antimicrobial factors at the site of infection.39 This interaction of activated leukocytes with platelets and plasma clotting factors in the innate immune response may contribute to the formation of thrombi (immunothrombosis).40
Dysregulation of NETosis can cause a state of hypercoagubility, triggering thrombosis. NETs promote thrombus formation by serving as a scaffold that activates platelets and clotting. This phenomenon has already been described in settings such as deep vein thrombosis, stroke, and myocardial infarction.41 In relation to COVID-19, NETs have been shown to contribute to the formation of microthrombi through platelet-neutrophil interactions in patients with respiratory distress.42
COVID-19 coagulopathy: a new entity?Studies based on autopsies of deceased patients from COVID-19 are the best way to discern whether multiorgan involvement in patients with COVID-19 is secondary to the multiple comorbidities present or is caused by a primary thrombotic disorder.6
The available literature shows discrepancies, since some authors did not find microangiopathy features in the organs, which is why it is attributed to associated comorbidities.43,44 In another serie of autopsies, the presence of generalized fibrinous microthrombi located at the level of the lung, respecting other organs such as the kidney and the skin.45 However, the presence of SARS-CoV2 has been reported in podocytes and renal tubules in patients with death due to respiratory failure. In addition to this finding in renal histology, structural damage attributable to other comorbidities was found, with the presence of microthrombi. A higher incidence of hypoxia and multi-organ failure was also seen, this suggests a mixed etiology of these alterations.46
Complement deposition in the lung has been reported, that suggest a systemic activation of both the classical and the lectin-based complement pathways. These deposits have also been seen in the microvasculature of the skin, both in purpuric lesions and in healthy skin tissue. These findings are consistent with multiorgan vascular disease.47,48
Microthrombi in pulmonary circulation are a frequent finding in autopsies for COVID-19. This phenomenon has also been previously described in autopsies of patients who died from H1N1 virus infection. These findings suggest the presence of an immune-mediated systemic coagulopathy that could be classified as the TMA associated with severe viral infection.49 The spectrum of TMA includes several diseases such as DIC, CAPS, and hemolytic uremic syndrome (HUS). All of them have in common an overactivation of platelets and the coagulation cascade.6 This situation of excessive activation of platelets increases the risk of thrombosis and subsequent consumption that can cause bleeding. But this rarely occurs in COVID-19 coagulopathy and in other TMAs.50 However, as we have explained above, complement activation could be involved in COVID-19 coagulopathy, as occurs in TMA syndrome.
Multiorgan involvement with complement deposition may occur in the context of a typical infection in CAPS and HUS (Shiga toxin). A high prevalence of antiphospholipid antibodies has been described in patients with COVID-19 (see below). In the case of HUS, SARS-CoV-2 has been seen in specific regions with alterations suggestive of microangiopathy47,49 suggests that this virus could be a trigger for coagulopathy as in the case of Shiga toxin in HUS.51 Distinguishing the different TMA syndromes is often difficult because there is an overlap in clinical and pathophysiological features like complement activation. These coagulopathies should be considered a spectrum disorder rather than independent diseases.6 As in the rest of coagulopathies, there is no single cause responsible for coagulopathy caused by SARS-CoV2. The sum of the events caused by the infection itself, the alterations in the complement cascade and coagulation, as well as the coexistence of other comorbidities favor a prothrombotic state.
Antiphospholipid syndrome and COVID-19Antiphospholipid syndrome (APS) is a systemic autoimmune disease characterized by the appearance of thrombosis or gestational morbidity (clinical criteria) in a patient with persistently high levels of antiphospholipid antibodies (aPL).52 The aPLs recognized in the classification criteria are lupus anticoagulant, anticardiolipin and anti-beta2-glycoprotein I antibodies of IgG and IgM isotypes. Presence of double or triple aPL positivity has been associated to higher risk of thrombosis.53 The aPL levels rise nonspecifically during acute infections.54 Therefore, to avoid false positives, the classification criteria indicated that to classify a patient with a thrombotic event as APS, there must be positivity of aPL in 2 determinations separated 12 weeks.55
There are three forms of APS: (1) APS associated with the presence of another autoimmune disease such as systemic lupus erythematosus, (2) an isolated APS form without other autoimmunity (primary APS), and (3) CAPS, the most severe form with multiorgan involvement, and evidence histopathology of multiple vascular occlusions. It associates poor response to anticoagulant treatment and a high risk of mortality.56
There are signs and symptoms not included in the classification criteria despite being very frequent, as livedo reticularis or thrombocytopenia. There are also extra-criteria aPL that have been associated with APS clinical events but are not included in the classification criteria. Among them the aB2GPI of IgA isotype, the anti-phosphatidylserine prothrombin (aPS/PT).57 A high prevalence of aB2GPI IgA antibodies has been seen in patients with kidney and heart failure on the transplant waiting list, and their presence has been associated with an increased risk of thrombosis and early graft loss due to thrombosis after transplantation.58,59 The origin of aPL is not yet well understood. From the first description of APS, it was observed the influence of microbial and viral agents influencing the production of aPL and an infectious etiology for this syndrome was postulated.60,61 It has been suggested that the mechanism of molecular mimicry between the β2GPI molecule and some molecular structures of various microorganisms may be present in the pathogenesis of APS.62
The mechanism by which aPL induces thrombosis is also not well understood. It is not well known. Meroni described the theory of the 2 hits, stating that the mere presence of aPL (first hit) is not enough to generate a thrombotic event. A trigger (second hit) is required to produce endothelial activation, such as complement activation, infection or surgery.63
Antiphospholipid antibodies in COVID-19The suspicion that there could be a relationship between APS and the prothrombotic situation of COVID-19 patients began with the observation of 3 patients with thrombotic events during admission, in which positivity was mainly observed for aPL of the IgA isotype (aCL and aB2GP1).64 Numerous reports of association of the presence of aPL with thrombosis in COVID appeared, such as cerebral infarcts in patients without a previous history of APS, many of them with double aPL positivity.65,66
After beginning to perform systematic aPL screening in COVID-19 patients, numerous studies emerged on the incidence of aPL in COVID-19 patients. The results surprised the scientific community, after showing high rates of presence of positive LA with a prevalence between 50 and 90% of COVID-19 patients67,68 especially among those with elevated aPTT, showing up to 91% positivity for LAC.69
Prevalence of aPL in COVID-19 patients“Criteria”-aPL prevalenceThe prevalence of IgG/IgM aCL and aB2GPI isotypes is lower than of LA. In fact, in patients with severe COVID-19, a positivity of approximately 15% has been shown.70–72 In addition, a large proportion of patients with more than one of the positive antibodies has been seen, the most frequent being double positivity for aPL (25–50%).72,73
There are no major differences in the prevalence of “criteria”-aPL, this could be due to the fact that the diagnostic kits are very well standardized and there is a great deal of experience. Although most studies have a very small sample, more studies are necessary in order to draw statistical conclusions.
“Non-criteria”-aPL prevalenceThe published prevalence data for these antibodies in COVID-19 patients are quite heterogeneous. Zuo et al. studied 172 hospitalized patients, measuring all aPL, they found that aPS/PT IgG 24% aCL IgM 23% were the most prevalent.74 In studies done on critically ill patients, high prevalences of atypical aPL have been shown, for example anti-annexin IgM antibodies were positive in 19%,72 or IgA isotype aPL, both aCL and aB2GP1 were positive in almost 30% of patients75 and its presence has been associated with the most serious cases of COVID-19 disease.76 In contrast, Borghi et al. analyzed all included extra-criteria aPL, in 122 patients finding prevalences<10% of extra-criteria aPL.71
Clinical associations with aPL in COVID-19Regarding the clinical association of aPL, something similar occurs with the prevalence study; there is no consensus on the pathogenicity of these antibodies. The different studies that have studied this association offer contradictory results.
Presence of associationZuo et al. showed that patients with aPL had more ARDS and lower glomerular filtration rate. In addition, they have shown that high titers of IgG isotype aPL induce the release of NETs by neutrophils, and showed the pathogenicity of these antibodies in an animal model.74
It has reported that COVID patients with multiple aPLs positivity had a significantly higher incidence of cerebral infarction compared to patients who were negative for aPLs (p=0.023), with IgA isotype antibodies being the most frequent.75
Absence of associationMany authors have not seen an association between aPL and thrombosis in COVID-19 patients; most of them study critically ill patients. However, all of them coincide showing a high prevalence of LA of almost 90% in critically ill patients.69,70,77 Despite not finding a relationship with thrombosis, it has been seen that IgG aCL is more prevalent in patients with more severe disease.78 Only one study analyzed the association of multiple aPL positivity, without showing worse results. This may be due to the low number of patients with several positive aPL, so it is not possible to make reliable statistics to demonstrate clinical association with thrombosis.77
Amezcua-Guerra et al. studied all classic aPL and extra criteria in 19 patients in ICU, being the IgM isotype the most frequent, they found no association to thrombotic events, attributing coagulopathy to severe infection.72 The correlation of aPL with domain I of B2GPI has also been studied, recognized by only 5% of patients. Thus, authors expose that these aB2GPI aPL are non-pathogenic antibodies directed against to other epitopes of the molecule.71 In a study carried out on 35 patients who died from COVID-19, with signs of coagulopathy and multiorgan thrombosis in more than 3 organs at autopsies, an 8% prevalence of classic and extra-criterion aPL was found but without clinical association.79
aPL in COVID-19 patients have also been compared with APS isolated and APS associated to other rheumatic diseases. Although COVID-19 is associated with more thrombotic events, aPLs seem to have no clinical relevance since their presence in patients with thrombosis is not different than seen in patients without symptoms.80
Is APS associated to COVID-19 a pathogenic entity?Given the high prevalence of aPL in COVID patients and its lack of association with the characteristic events of APS, it has been suggested that these aPL are actually false positives.
“Criteria”-aPLThe origin of this possible false positivity would be different in LA and in the aCL/aB2GPI. It is postulated that LA positivity is due to anticoagulation, a treatment that is administered to almost all patients with severe COVID-19 infection.81 To avoid this high proportion of false positives, it is recommended that the extraction to determine LA be done at least 12h after the heparin dose.82 This higher prevalence has also been attributed to the fact that most patients are elderly,83 therefore, it is mandatory to develop new studies which include control groups of similar ages to assess the true incidence of LA. Another possible element of uncertainty would be the interference with high levels of C-reactive protein, this phenomenon is very frequent in COVID-19 patients.84 Therefore, LA positivity should be interpreted with caution if performed in these patients.77
Analyzing aCL/aB2GPI antibodies of IgG and IgM isotype, the most common are aCL, however, the positivity of these antibodies has been associated with infectious diseases on numerous occasions85 and with uncertain pathogenicity.86 It must be noted that aPL of COVID-19 patients very rarely (5%) recognize domain I of B2GPI,71 which is the region of the molecule most commonly associated with pathogenicity.87
The clinical association of IgM isotype aPL with thrombosis is quite controversial, some authors have found no association between aPL IgM isotype and thrombosis.88 False-positive results can occur when coexist with other causes of thrombosis in as atherosclerosis in the elderly difficult to interpret, therefore some authors do not recommend screening.89 However, the presence of IgG and IgA isotypes at the onset of symptoms is possible because they were already performed prior to infection. The switching class from IgM to IgG or IgA is a process that requires a long evolution time, so it is unlikely that they are antibodies generated during infection. Although infection could increase the preformed IgG and IgA antibody titer due to secondary immune system activation.
“Non-criteria”-aPLIn the case of “non-criteria”-aPL, not only influences the uncertainty inherent to other aPLs but also should be added the controversy regarding their validity, and the lack of standardization of the tests. Therefore, it implies a great heterogeneity in the results depending on which system is chosen to detect these antibodies.90 Currently the tests based on solid phase (ELISA) show more reliability in their results. On the contrary, with the use of semisolid systems based on antigens adhered to beads, a large proportion of false negatives can be obtained.71,91
The problem of diversity of results is especially frequent in aPL of IgA isotypes. Interestingly, this isotype has been shown as the most prevalent in a study,75 although other detect levels comparable to those of the general population.71 It should also be noted that most studies on aPL in COVID-19 have been conducted with small samples, and a second confirmatory determination is very rarely made after 12 weeks. When has been evaluated, it has been shown that they are transient and become negative over time.77
In summary, to definitively determine the possible association between aPL and COVID-19, rigorous studies with larger cohorts should be carried out. Blood samples must be extracted at least 12h after administering heparin-type anticoagulants. Antibody titers evaluated with solid phase techniques sufficiently contrasted (especially in extra-criteria antibodies) and systematically confirm the results with a second determination carried out at least 12 weeks after the first.
Treatment of APS in COVID-19The hypercoagulability associated with COVID-19 appears to be directly related to the severity of the disease and to a worse prognosis for patients. Therefore, therapeutic decisions often include the use of therapeutic anticoagulation in high-risk individuals.92
Current data recommend the use of prophylactic anticoagulation with low molecular weight heparin in hospitalized patients with COVID-19. It should be taken into account that regardless of the thrombotic association of aPL, antithrombotic prophylaxis should be done due to the high thrombotic risk as a consequence of multiple factors of hyperinflammation, platelet activation, and endothelial dysfunction. So its positivity should not change this practice.93
Current data suggest that anticoagulant treatment improves the results. However, despite anticoagulation, more thrombotic complications were significantly observed compared to non-COVID-19 ARDS patients (11.7% vs. 2.1%, p<0.008).67 This situation has a certain parallelism with the CAPS.94 In COVID-19, possibly higher anticoagulant doses should be administered and associated with other adjuvant treatments, making an analogy with the therapeutic approach of CAPS, whose treatment is based on the combination of heparin, corticosteroids and plasmapheresis.95 In this way, anticoagulation and corticosteroids have shown good results in these patients, although there are very few data on plasmapheresis. Perhaps it could bring benefits in the subgroup of patients with COVID-19 with high titers of aPL.74
Final reflections on APS and COVID-19The data provided by the literature show that there is a coagulopathy associated with COVID-19 that encompasses a spectrum of alterations that suggest an immune-mediated mechanism reminiscent of APS, and its most severe form, CAPS.
In this way, the early introduction of anticoagulant treatment led to an improvement in the prognosis of these patients. In addition to APS, many similarities have also been found with other subtypes of TMA. A high prevalence of aPL has been reported in COVID-19 patients, especially in older patients with a worse clinical course, although these alterations can act as confounding factors. For this reason, there is great controversy about the clinical association of these antibodies with thrombotic events. The current results do not allow a statistical relationship to be established.
It is important to take into account that most of the studies show important methodological shortcomings that make difficult to establish correct interpretation of the aPL. Especially the absence of control populations or, in the cases in which they are included, these controls are not comparable because they tend to be much younger than the patients studied. It is known that infections cause temporary elevation of aPL, therefore, the classification criteria require repeating a second determination at 12 weeks, which is not performed in almost all studies. Another no less important deficiency is the heterogeneity of the methods for determining aPL, with large discrepancies in prevalence depending on the different diagnostic systems used. Sufficiently contrasted solid phase tests should be used to minimize this problem.
The evidence of the results suggests the determination of the most important extra-criteria aPL (IgA isotype of anti B2GPI and aPS/PT), since they are the most prevalent aPL, and should be incorporated into aPL studies to assess COVID-19 coagulopathy.
Despite the controversy that exists in the clinical association of aPL, the analysis of the results suggests that in most patients, aPL would not be the direct cause of the procoagulant situation in COVID-19 coagulopathy. This is because it is possibly a multifactorial coagulopathy, in which various mechanisms participate including aPL, in an additive way. It is necessary to evaluate whether in the context of the infection there are alterations in the levels of the molecules related to the coagulation processes, such as elevations of procoagulant molecules (such as proteases) or deficiencies of anticoagulant molecules (such as serine proteases, protein C, etc.) or phospholipid-binding proteins. These alterations could be further favoring the generation of a prothrombotic state in these patients. Despite the existence of similarities with various microangiopathies, we are probably facing a new coagulopathy secondary to SARS-CoV2 infection. New studies are needed to better explain this new immune-mediated phenomenon.
It is mandatory to carry out systematic studies on the presence of aPL in large groups of COVID-19 patients, assessing both the initial prevalence and the temporal evolution to confirm which ones remain positive and the possible relationship of the presence of aPL with the appearance of complications in the patients not only in convalescence but also in the medium and long term.
Conflict of interestThe authors declare they have nothing to disclose regarding conflict of interest with respect to this manuscript.